As we continue in our familiarization with Propane and Natural Gas, we must realize that with both fuels, the characteristics of the flame are important to safe and efficient operation of all appliances using the product. As oil companies transition into the gas market, new appliances will now have to be considered. With oil, it was pretty much heating and hot water; But with gas, we have many more appliances involved, all of which will burn the fuel. This includes everything from gas ranges, dryers, space heaters, unvented heaters, fireplace inserts, pool heaters and outdoor grills—in fact, there are unlimited uses for these fuels. There are also new safety considerations, such as gas leaks and how to handle those (we will be discussing these in later articles). With propane, another concern is out of gas calls and the required pressure tests and leak tests that must be performed when dealing with those calls (these will also be covered in later articles).
The two fuels, because they are already gases, are ready for burning with reduced residuals and usually no sooting. All other fuels require some change of characteristic before they will burn, including coal, wood and even oil; all must turn to a form of gas before they will burn. In the case of oil, this is accomplished with pressure to atomize the oil through the nozzle. That combustion gives off visible light, detectable by the CAD cell for safe operation. But with propane and natural gas, there is very little visible light, therefore a CAD cell would not be able to detect the gas flame. However, the gas flames do give off infrared and ultraviolet, and in particular on commercial and industrial applications, we do have ultraviolet flame detection systems, allowing burners to operate safely. These devices are too costly to be used on gas residential systems. With that in mind we have to have other safety devices for flame detection and safe operation. We will be addressing those in future articles.
The concern with both propane and natural gas is to accomplish burning with little to no carbon monoxide (CO). The ANSI (American National Standards Institute) standards for heating and water heating is 0.04% (400 Parts per Million in a flue sample). For gas ovens, it is 0.08% (800 PPM). These are really too high—we strive for under 100 PPM on heating and hot water systems. On gas ovens, we would like to be at 25 PPM or below—the lower the better. As for CO levels in the living environment, EPA states 9 PPM for residential living spaces. OSHA and NIOSH for work environments specify 35 to 50 PPM on a time weighted average of 8 hours.
Let’s cover some of these characteristics and how we accomplish them.
FLAME CHARCTERISTICS
The proper gas flame is a soft blue flame, which is stable, not lifting or floating around.
The three distinct cones as shown in

Figure 1 Click on image for larger view
Figure 1 should be visible. There should be no yellow in the flame as that is a characteristic of carbon.
The outer cone of the flame (the hottest part of the flame) should not be touching any surfaces. When the outer cone “impinges” on cooler surfaces, such as boiler sections or furnace heat exchangers, carbon deposits are formed and carbon monoxide is present.
The outer mantle may touch surfaces, as it is simply the products of combustion.
When looking at the main burner flame as in Figure 2, the same distinct cones should be visible. There may be times when “orange flecks” appear in the flame. This is caused by stirring up dust when in the vicinity of the burner or disturbing the burner and loosening a little rust or dust. This is of no concern as it will burn off. The only concern is for luminous yellow flames.
If, for some reason, the hotter part of the gas flame (outer cone) should touch a cooler surface, this is called “impingement”. In Figure 3, this is illustrated. This shows that carbon deposits would accumulate on the surface of heat exchanger or boiler surfaces.

Figure 2: Click on image for larger view
There would also be another class of compounds called “Aldehydes” formed due to incomplete combustion. While carbon monoxide is odorless, aldehydes have a sharp, penetrating odor. The odor of aldehydes differs from odorants added to the gas for detection and the two should not be confused. The absence of aldehydes does not assure that carbon monoxide is not present in the flue products. However, if the odor of aldehydes is present, then carbon monoxide will almost always be present. Aldehydes, much like carbon monoxide, are toxic.
Combustion
It is important to understand the basics of combustion. In all aspects of operation, proper and safe combustion is of primary importance. There are many factors which can affect good combustion. It is important to understand that beyond what we will discuss here, there are very complex issues relative to combustion which need to be considered.

Figure 3: Click on image for larger view
The Meaning of Combustion
All matter is made up of basic substances called elements. One of the most common elements on earth is oxygen. About 20 percent of our air is oxygen. Many materials react with oxygen, a process called oxidation. Rusting of metals is a common, everyday form of this process. Heat must be added to some oxidation reactions for them to take place and continue. Heat is given off in others. When oxygen acts with a substance to produce large amounts of heat rapidly (and usually light) the process is called combustion or burning.
With enough air present, fuel gases will burn in their normal state. Solid or liquid fuels, on the other hand, must be changed to a gas first before they will burn. Usually heat is required to change liquids or solids into gases
Requirements for Combustion
Three things needed for combustion to take place are shown as a combustion triangle in Figure 4: fuel, oxygen (air) and heat (temperature) all must be present. Otherwise, burning will not start or will not sustain itself after it starts. Take away any one of the three and burning will stop. This fact can be shown by considering ways to put out a wood fire. If all of the unburned wood (fuel) is dragged a way from the fire, the fire will soon go out. A heavy blanket may be thrown over the fire. The fresh supply of air (oxygen) is cut off, smothering the fire. Foam type fire extinguishers work in the same way, cutting off the air supply to a fire. Water thrown on the fire chills the wood. Reducing the temperature below the kindling point causes the fire to go out. Of course, heat is needed to start the fire in the first place. The same holds true for a gas “fire”. Some source of heat is required that will raise the temperature of the gas to its ignition temperature, which is (1,100° to 1,200° F).
In burning gas, all three elements of fuel, air and heat must be present. Gas alone will not burn. Air which supplies oxygen is needed as well. An open flame (pilot), spark ignition or even a hot surface igniter may be used to ignite the gas in gas appliances. Then the gas flame itself provides the heat need for sustained combustion.
Basic Chemistry of Combustion
Combustion of gas is a chemical reaction between fuel gas and oxygen. Figure 5 illustrates the case of the combustion of methane. The basic elements of common fuel gases are hydrogen (H) and carbon (C). When hydrogen burns, water vapor is produced. The water molecule, represented by the symbol H2O, consists of two hydrogen atoms and one oxygen atom. Complete burning of carbon in fuel gases forms carbon dioxide. Its symbol is CO2 and it has one atom of carbon and two atoms of oxygen in each molecule.
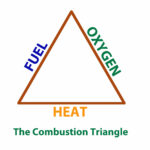
Figure 4: Click on image for larger view
A fuel gas (methane, for example) and oxygen react with each other in burning, so are called reactants. Water vapor and carbon dioxide formed by that burning are called products of combustion.
In Figure 5, the reactants are methane (CH4) and oxygen. Two molecules of oxygen are required for each molecule of methane. The products are carbon dioxide and water vapor. Burning one molecule of methane produces one molecule of carbon dioxide and two molecules of water vapor. The total number of each type of atom in the reactants must equal the total number of the same type of atom in the products.
Figure 5 also shows complete burning of methane with exactly the amount of oxygen required. This basic model of the burning process should be understood for three reasons:
First, it shows that only harmless carbon dioxide and water vapor result if a fuel gas is completely burned.
Second, it shows exactly the amount of oxygen needed to completely burn a given fuel gas.
Third, it shows that definite amounts of products are formed in completely burning a certain quantity of a fuel gas.
The model shown in Figure 5 is expressed in terms of molecules. This figure also can be expressed in terms of cubic feet of the gases involved, substituting cubic feet for molecules. Two cubic feet of oxygen are needed to completely burn one cubic foot of methane. This process will form one cubic foot of carbon dioxide and two cubic feet of water vapor. Normally, of course, air containing oxygen rather than pure oxygen is used to burn a fuel gas.
Complete Combustion
Figure 5 shows an ideal model of complete burning of methane with oxygen. In gas appliances, air, rather than pure oxygen, is used to burn the gas. Air contains about 20 percent oxygen and 80 percent nitrogen.

Figure 5: Click on image for larger view
I am sorry to report that conversions to gas continue to climb in many cases, just for the sake of cost of fuel. That, in my estimation and experience, is not the primary reason customers should change fuels. You might think I, coming out of the gas industry, would be gung ho for gas all the way, but that is not really the case. I am a believer in fair competition and insuring that customers continue to have a choice.
We should all endeavor to point out to our customers the plusses and minuses of fuel and equipment choices. The truth is, we are in the energy business today and we need to look to diversity as our offering to customers. It is also important that we all tell the truth about the pros and cons of our fuels, equipment and the servicing of all that. I hope my articles here will help to unite as to a common cause, and that is the safety, comfort and efficiency of customer’s equipment and homes. So with that in mind, we’ll continue our presentation on gas fuels.
Physical Characteristics of Fuel Gases
Natural Gas
Petroleum gases are hydrocarbons—a chemical structure of hydrogen and carbon. Natural gas is primarily methane (CH4) and ethane (C2H6), but it will typically contain a number of heavier hydrocarbons when it leaves the well. Among these are propane and butane (the prime constituents of LP-gas), pentane, hexane, septane, octane and decane (the natural gasolines).
Natural gas right from the well will also contain impurities such as water, carbon dioxide and helium. The volume of heavier hydrocarbons is called the “wet gas” content. These heavier hydrocarbons are normally removed from natural gas by condensation and are used in the manufacture of LP-gas and gasoline. Impurities are also removed during refinery processes and the resulting “dry” gas is the natural gas commonly marketed.
At temperatures above –260°F at atmospheric pressure, natural gas occurs as a gas. It is normally transported from the source to the consumer in a gaseous state by pipeline. Recently, methods have been developed to transport natural gas as a relatively low pressure, low temperature liquid by ocean-going tanker. To accommodate periods of peak demand, natural gas may be stored as a pressurized gas or as a pressurized low temperature liquid. Natural gas is costly to liquefy because very low temperatures are required. The use of liquefied natural gas as a motor vehicle fuel is possible only with thick-walled storage tanks, well insulated to maintain the fuel in a liquid state. As a practical matter, natural gas is only liquefied to make bulk storage and transportation possible.
The fuel is lighter than air, weighing from 56% to 79% of the weight of an equal volume of air. The specific gravity of natural gas is, therefore, within the range of .56 to .79. Released into the atmosphere, it will normally rise and mix readily with air.
When burned efficiently, one cubic foot of processed or “dry” natural gas will normally produce 900 to 1,200 British Thermal Units (Btus) of heat, depending on the exact specific gravity. This value is called the gross heating value of natural gas and is an important factor in the design and operation of heat producing appliances.
Natural gas is colorless, odorless, and nontoxic. Although nontoxic, it can displace oxygen in the air and cause unconsciousness and death through asphyxiation, due to lack of oxygen. To aid in the detection of leaks, odorants are normally added to natural gas before distribution. Mercaptans are sulfur compounds commonly used as odorants. Although added in small amounts, they provide an effective means of detecting gas leaks.
LP-Gases
Propane (C3H8) and butane (C4H10) are chemical compounds of hydrogen and carbon (hydrocarbons) which occur in “wet” natural gas and in crude oil. These heavier gases are removed from “wet” natural gas along with other impurities in order to produce “dry” natural gas for distribution. In oil refineries, these gases are produced in the process of manufacturing gasoline and other petroleum products.
In 1971, approximately 74% of LP-gas marketed was produced at the well from “wet” gas; the remaining production came from refineries. The ratio of production from wells and refineries varies from time to time, but in general the newer gasoline-making processes are yielding a higher percentage of propane. At normal temperatures and atmospheric pressure, both butane and propane occur in a gaseous state. Pure butane will condense to form a liquid at temperatures below 32°F when it is at atmospheric pressure. Both gases, however, will condense to form a liquid at normal temperatures and relatively low pressures.
Processed butane is normally 93% butane and 7% propane; processed propane is normally pure. LP-gas is distributed as a liquid by pipelines, rail cars, tanker trucks and tanker ships and barges. It is normally stored in pressurized tanks. Transportation to the consumer is usually by bulk truck. Storage at the point of use is in small tanks or cylinders, as a liquid under pressure.
Both butane and propane gases are heavier than air with specific gravities of 2.00 and 1.53 respectively. Neither propane nor butane gas will rise and disperse as quickly as natural gas when released into a nonventilated area. Because both gases are heavier than air, LP-gas will normally settle in calm air to form pockets, or it will hover around the source of the leak. With turbulence of ventilation, LP gas dissipates almost as quickly as natural gas.
Propane has a gross heating value of about 2,500 Btu/cubic foot; butane has a gross heating value of about 3,225 Btu/cubic foot. A given volume of LP-gas, therefore, will produce two and a half to three times the number of Btus as an equal volume of natural gas. This is an important consideration in the conversion of appliances from one fuel gas to another.
Butane and propane are colorless, odorless, and nontoxic. LP-gases also can displace oxygen in the air and cause unconsciousness and death through asphyxiation. Again, to aid in the detection of leaks, odorants are normally added to LP-gas before distribution to the consumer. In nonventilated areas, LP-gas leaks are more difficult to detect than natural gas leaks because LP-gas does not rise and disperse in air as readily as natural gas.
Summary
Petroleum gases utilized in heating appliances are natural gas and liquefied petroleum gas. Natural gas occurs naturally as a gas and requires minimal refinement. LP-gas occurs in “wet” natural gas and crude oil and must be extracted and refined before use. Natural gas is normally distributed in pipelines as a gas; LP-gas is usually transported under pressure as a liquid. Both gases are colorless, odorless, and nontoxic in their natural state. Natural gas is lighter than air and will rise and disperse. LP-gas is heavier than air and will settle and hover around the source of a leak.
Comparison of Fuels
When a comparison is made of fuels as to which one is the better fuel for heating, it has always been that oil comes out on top. The reason is the higher BTU per gallon as compared to the other fuels. The examples given are:
1 Gallon of #2 Fuel Oil = about 140,000 BTUs
1 Gallon of #2 Fuel Oil = about 1.4 CCF Natural Gas
CCF Natural Gas = about 0.7142 Gallon #2 Fuel Oil
Fuel Cost Conversion Formulas:
Cost per Gallon Fuel Oil x 0.7142 = about cost per CCF Natural Gas
Cost per CCF Natural Gas / 0.7142 = about cost per Gallon #2 Fuel Oil
Examples:
If Fuel Oil costs $1.50 per gallon, the BTU equivalent cost of natural Gas is $1.50 x 0.7142 = $1.07 per CCF
If Natural Gas costs 1.00 per CCF, Fuel Oil would have to cost ($1.00 / 0.7142 = $1.40 per Gallon) to match the BTU cost of Natural Gas.
In the past, when comparisons were made of Natural Gas or Propane to oil relative to heating equipment, it was always atmospheric gas heating equipment. That equipment was compared to the oil power burner type equipment. When this comparison was made, oil always came out on top when efficiency numbers were discussed.
I am not a big fan of discussing efficiency as related to numbers such as 75% or 80% efficient. I will, as my articles continue, explain that. The reason oil was more efficient when looking at combustion efficiency numbers was that it was compared to gas equipment using room air for combustion (atmospheric). The oil power burner needed less excess air, usually around 25% as compared to the atmospheric gas equipment, which needed 40% to 50% excess air in order to burn safely and efficiently. Years ago, nobody was too concerned about that as fuel was cheap and most of our equipment on both sides was vented into chimneys. The interesting thing about all of that is that when the oil power burner was replaced by a gas power conversion burner, the gas efficiency numbers then ran right alongside the oil simply because not as much excess air was required. In some cases, the efficiency slightly exceeded the oil numbers.
Controlling excess air makes the difference when looking at this from a combustion perspective. In my many years in the gas industry, I have been involved in one way or another with over 3,000 conversions from either coal or oil to gas using a power gas conversion burner. In all cases, when the conversion was completed, we strived for a final efficiency number of 75% and in most cases, were able to accomplish that.
The chart below compares the average characteristics of common fuel gases. Notably, when we talk abo

Click on chart to enlarge
ut maximum flame temperature in air, the scale runs Butane as hottest, then Propane and finally Natural Gas? What place does # 2 oil have? Well, it is the highest, at about 3,820°F.